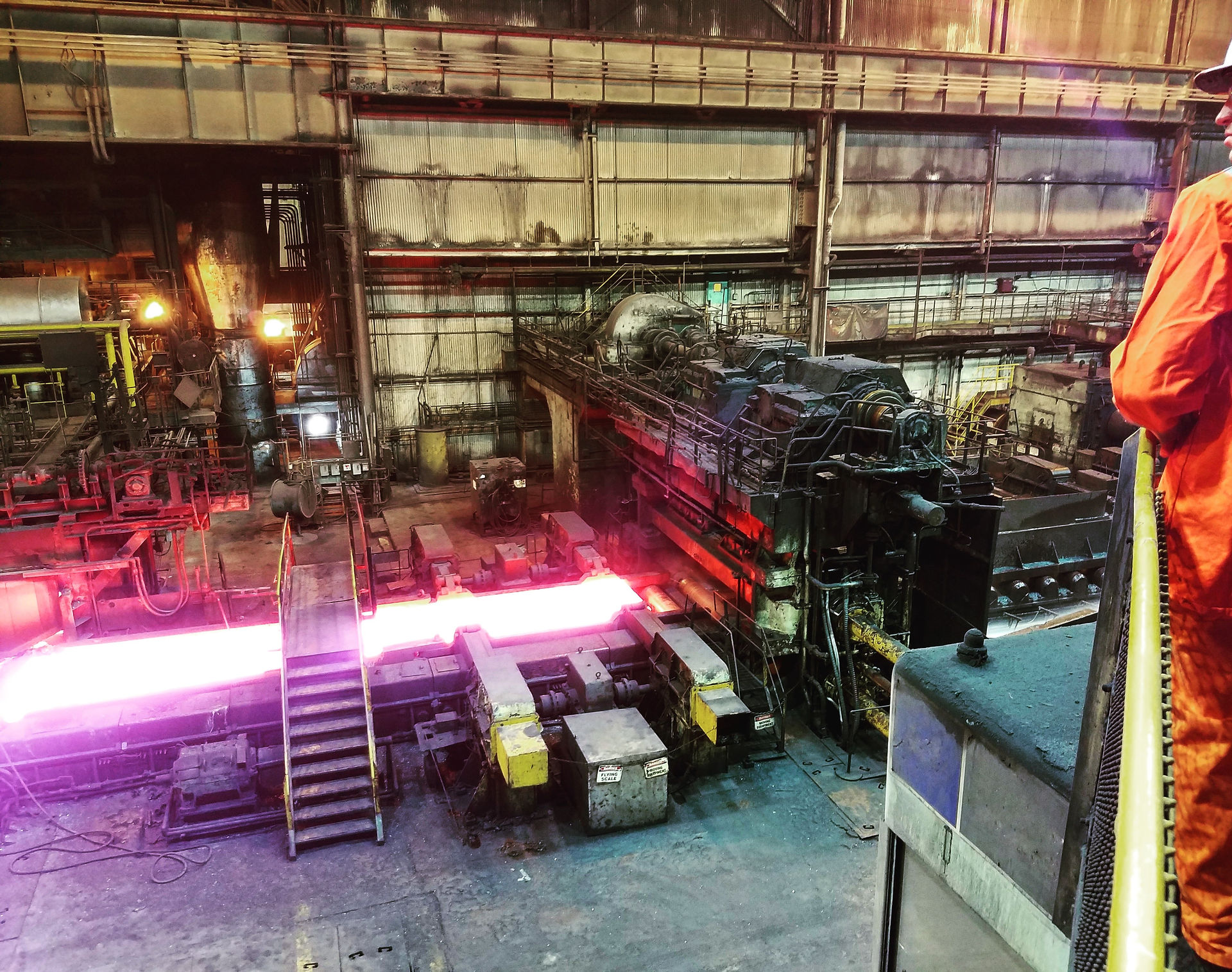
steelmaking 101
BOF vs EAF
ALLOYING
integrated vs mini
Integrated Steel Mills
-
Continuous operation: very costly (can be over $1 million) to stop and start
​
-
Very expensive to reline blast furnaces (about $100 million)
​
-
Low residuals leads to higher quality and chemistry control
​
-
Production as high as 10,000 tons a day
​
-
Large volume markets required
​
-
About 250 tons/heat
"Mini" Steel Mills
-
Easy to start and stop; very flexible and more easily adapts to slow markets
​
-
Ideal for batch operations
​
-
Quality restrained by higher residuals, a byproduct of using scrap
​
-
Low capital investment
​
-
Very dependent on the price of scrap
​
-
Lower capacity, but ability to be closer to markets for reduced freight
​
-
About 150 tons/heat
the hot strip mill
Once the steel has been cast into a slab, it needs to be rolled down to the ordered thickness. This happens at the hot strip mill.
​
At integrated mills, where slabs are cast 8 - 10" thick, slabs are transported from the caster to the slab yard to cool. They then must be brought back to temperature in a reheat furnace (about 3 hours at 2300F) before they can be rolled.
​
At mini mills, the process is continuous. 2 - 4" slabs coming out of the caster immediately continue into a tunnel furnace; this holds their temperature so a drastic amount of heat isn't lost in between casting and rolling. From the furnace, the slabs are charged directly into the hot strip mill.
At the hot strip mill, the slab is carried on a series of motor-driven rolls. Since steel forms abundant scale (an iron oxide that forms when the steel surface contacts oxygen) at high temperatures, the first step in the process is to descale the slab. If the scale "jacket" around the slab passes through the rolling stands, it will get pressed into the surface of the steel and cause imperfections or defects (see here). To descale, high-pressure water is sprayed across the surface of the slab to break apart and blow off the brittle scale.
​
The slab then passes through a series of roughing stands that reduce the bulk of the thickness. Because scale develops quickly, there is high-pressure water at each roughing stand to remove newly formed scale.
Once the slab (now called the transfer bar) has finished passing through the roughing stands, it continues on to the finishing train. The finishing train is a set of 5 to 7 stands arranged in tandem, and it's here that the transfer bar is reduced to its final thickness.
​
As the transfer bar is fed into the finishing train, it begins to move faster and faster as the thickness is reduced. To keep the mass flow constant, "loopers" lift between the stands; this allows for some of the steel strip to gather before entering into the next stand. After passing through the last stand, the steel strip is at its final thickness, and continues on to the cooling portion of the hot strip mill. Very thin gauges can exit the finishing mill at over 40 mph.

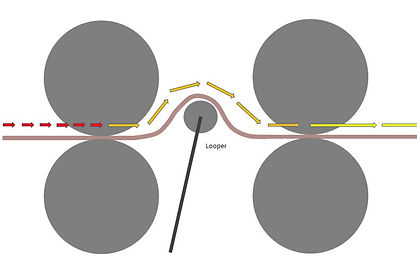
The cooling tables are situated directly after the finishing train so that the desired microstructure can be locked into place immediately after rolling. Here water is poured onto the strip to cool the steel from 1200 - 1800 degrees to only a few hundred degrees. After cooling, the strip is coiled at the downcoiler and the new hot rolled "band" is formed.
alloying
Steel is comprised mostly of iron, but the other elements present in small amounts play large roles.
​
The properties of steel can be manipulated using chemistry, but improving one property usually comes with a trade-off. For example, some of the strongest steels, martensitic steels, have excellent strength but little ductility. Choosing the ideal properties of steel for a certain application is all about balance - the desired properties are maximized, but not at the expense of the overall product.
​
This is a driving force behind much of the product development in the steel industry today; TRIP steels, for example, offer high strength with improved ductility.
​
Nonmetals
​
> Carbon
​
Carbon is the fundamental hardening element of steel. As iron cools from red-hot to room temperature, it undergoes a phase change that affects the solubility of carbon. As the temperature drops and the solubility decreases, the atoms separate into two phases: low carbon "ferrite" and high carbon "cementite." Together, they are called "pearlite." This layered structure is the key - pearlite has a tensile strength about triple that of ferrite, and about 24 times that of cementite.
​
> Phosphorus
​
In small amounts phosphorus can increase strength and hardness, but when present in higher amounts (>0.05%) it can reduce toughness and ductility.
> Sulfur
​
Sulfur combines with iron to form iron sulfides, which are present as inclusions in steel. Inclusions can serve as initiation sites for cracks and can reduce fracture resistance. Sulfur is normally regarded as an impurity, but one beneficial effect of sulfide inclusions is the improvement of machinability (related to the reduced fracture resistance).
​
> Silicon
​
Silicon is used a deoxidizing agent, as it combines with oxygen to form silicon dioxide (silica). It also promotes coarse (large) grains. Steels that contain high amounts of silicon may be at risk of developing silicon streaks. If a steel contains a "reactive" amount of silicon, it may cause an undesirable amount of zinc to adhere to the surface during galvanizing; this is addressed in ASTM A385.
​
> Nitrogen
​
Nitrogen increases hardness in very low carbon steels.
​
​
Metals
​
> Manganese
​
Manganese increases the hardenability and strength of steel. It also forms manganese sulfide particles, which prevent the sulfur from forming detrimental iron sulfides.
​
> Aluminum
​
Aluminum has a strong affinity for oxygen, and it is used to "kill" the steel. The solubility of oxygen in steel decreases as the steel cools, so excess oxygen can cause gas to bubble out. This is particularly dangerous during casting, as the oxygen gas may cause a mold breakout. Deoxidizing is called "killing" the steel because it calms and quiets the liquid steel. Most of the aluminum oxides that form float out of the steel as slag, but some of the unreacted aluminum remains in the steel and ultimately leads to very fine grains (a strengthener).
​
> Copper
​
Copper improves corrosion resistance and also offers strength through precipitation hardening. Because copper is not easily oxidized it is difficult to remove from steel; this presents problem for mills that entirely melt scrap (like mini mills). At typical processing temperatures, copper does not dissolve in the scale that forms on the surface of the steel. Instead the copper is forced back into the steel, where it concentrates at the surface. While the temperature is still above its melting point, copper will penetrate the grain boundaries and cause tearing as the steel is rolled. This is called "hot shortness."
​
> Nickel
​
Nickel improves impact strength and toughness, along with improving corrosion resistance.
> Chromium
​
Chromium increases hardenability and abrasion resistance, and unlike nickel, performs well at high temperatures. Chromium creates tightly adherent oxide layers on the surface of steel, and due to this feature it is the key element in stainless steels (>12% chromium). This layer protects the steel in many corrosive environments.
> Molybdenum
​
Molydenum improves hardenibility and high temperature strength.
​
​
Metalloids
​
> Boron
​
In low carbon steels, boron combines with nitrogen to form boron nitride, which softens the steel. In medium and high carbon steels, boron increases hardenability.
​
​
Microalloys
​
> Columbium/Niobium
​
Columbium is a strong grain refiner, which effectively raises the yield strength of the steel.
​
> Vanadium
​
Vanadium forms carbides and nitrides, and improves strength and toughness.
​
> Titanium
​
Titanium also strengthens by forming carbides.
​
​
And also...
​
> Oxygen
​
In its basic form, iron is bonded to oxygen - iron ore is an iron oxide. Whenever iron is exposed to oxygen, it will oxidize, forming slag or scale. Strong deoxidizers are added to steel to remove oxygen, but it is nearly impossible to remove all traces of iron oxides within the steel.
testing
Mechanical properties of steel determine how it will perform under loading. To be certified to a certain grade or specification, the steel must meet the minimum requirements for mechanical properties outlined in the specification. Testing usually consists of measuring the strength, elongation, and hardness, but critical applications may demand impact testing or other further qualifications.
​
Most end uses require that the steel meet a minimum strength, either a yield strength or a tensile strength.
​
- Yield Strength
​
The "yield strength" is a measure of a material's elastic response. When a stress (force/area) is applied to the material, the bonds that connect the atoms actually being to stretch. If the stress was removed, the bonds would return to their original state and there would be no permanent change in the material. The yield strength measures the maximum stress a material can withstand without the bonds actually breaking. Once the bonds have broken, new bonds form between newly adjacent atoms, and permanent deformation has occurred in the material.
​
​
- Tensile Strength
​
If the stress continues to increase beyond the yield strength, the material will "plastically deform." The bonds will continue to break and reform with new atoms, and more and more permanent deformation will occur. Eventually the material won't be able to form new bonds, and as the stress continues to increase, the material will fracture. This is the "tensile strength" - the ultimate stress a material can withstand before fracture occurs.
​
​
- Elongation
​
The amount of plastic deformation that occurs before fracture is the "elongation." If a material is ductile, it will visibly deform (or stretch) before it fractures. This is very important for almost all applications; if the steel will be formed, it must be ductile enough to bend without breaking. And consider the factor of safety allowed by ductile failure - if a crane boom is loaded to its ultimate tensile strength, a sudden, unexpected brittle fracture could be very dangerous. Steel that deforms under the loading gives warning that it is being stressed to its limits.
​
​
The yield strength, tensile strength, and elongation are determined using a tensile test. A small sample called a dog bone is cut from the steel and loaded into a tensile tester. The machine grips the ends of the dog bone, and as it pulls them apart it measures the stress on the sample until the sample breaks. The output is a stress-strain curve, and it's a plot of the loading vs deformation that allows the yield strength and ultimate tensile strength to be calculated. The elongation is determined by measuring the difference between the original gauge length (a two inch distance marked onto the original dog bone) and the final gauge length (the distance between the two markings after the test has been completed).
​
​

Engineering Stress (ksi)
Engineering Strain (%)
- Hardness
​
Hardness is a measurement of a material's resistance to compressive deformation. Instead of pulling, like in a tensile test, an instrument presses into the surface of the material and measures either the distance traveled or the size of the indentation. Different types of hardness tests include Rockwell A, B, and C, Brinell, and Vickers.
​
​
- Impact Testing
​
Also called a Charpy test, an impact test measures the toughness of a material. Certain metals undergo a "ductile to brittle transition," which means that at lower temperatures they exhibit brittle fracture when normally (at higher temperatures) they are ductile. The higher the impact test results, the more energy absorbed, and the more ductile the failure.
​
A real life example of the importance of the ductile to brittle transition is the Liberty Ship failures. When the ships were placed into cold waters, stress risers lead to brittle, catastrophic cracking.
​